1. 引言
燃气储存、输运设备因老化、腐蚀、第三方破坏等容易发生泄漏事故,一旦泄漏遇到火源,容易导致喷射火事故。燃气泄漏喷射火是燃气输运或储存过程中由于设备破损导致气体泄漏,遇点火源或因高压泄漏气体摩擦起火引起的特殊火灾现象。喷射火因高温、辐射等易造成严重事故后果,并容易引燃相邻可燃物、导致火灾爆炸等二次事故,引发多米诺效应 [1] 。许多研究已经研究了无撞击自由喷射火的几何结构和热辐射。然而有障碍物限制下的喷射火研究较少。在撞击过程中,火焰延伸面积和沿目标表面的温度分布对确定对流热非常重要 [1] 。因此,前方垂直障碍物限制下的泄漏燃气喷射火特性研究对于减少油气管道泄漏事故中的喷射火造成的损失有着重要的现实意义。
Wang [2] 证明了Fire FOAM软件可以是预测高压氢/甲烷射流火灾风险分析的可靠工具。Xiao [3] 使用CFD程序中的GASFLOW-MPI软件研究了通风室中氢气射流火灾的燃烧动力学,包括火焰熄灭引起的氧消耗脉动过程和新鲜空气流入引起的自燃过程。Zheng [4] 研究了压力容器意外泄漏引起的射流火灾事故的危险特性,并提出了一个综合多相可压缩射流火灾扩展模型。Mercedes [5] 研究了丙烷垂直射流火焰的轴向温度分布,结果显示温度从火焰底部增加达到最大值,然后在顶部区域下降。二次多项式表达式很好地描述了温度随着火焰中心线位置的变化趋势。Hu等 [6] 研究了低压大气中垂直喷射火焰的轴向温度分布,并提出了连续火焰区域、间歇火焰区域和浮力羽流区域无量纲理论能较好地表示垂直浮力湍流扩散喷射火在两种压力下的轴向温度分布。在水平射流火焰的火焰形状、温度场和热辐射强度方面,Gopalaswami等 [7] [8] 、Zhang等 [9] 和Imamura等 [10] 研究了水平射流火焰的长度和温度衰减模型。Zhou等 [11] 基于线源理论发展了一个热辐射模型,用于预测水平射流火焰的辐射强度,而Brennan等 [12] 研究了湍流强度和长度尺寸对火焰长度和宽度的影响。Houf等 [13] [14] 发现障碍墙在抑制射流火焰、减少点火超压和火焰辐射热流方面是有效的,而Schefer等 [15] 对高压氢气射流火焰与障碍墙的相互作用进行了实验研究。Tao等 [16] 研究了受三面垂直墙限制的垂直射流火焰的空气盘旋强度和火焰形状。
现有研究侧重于研究喷射火几何特性和计算模型模拟,涉及到障碍物限制下的喷射火特性研究较少。本研究分析了不同喷嘴–板间距、气体泄漏速率对射流火焰燃烧特性的影响。研究结果对国内外燃气输运和储存过程中火灾事故现状和研究都有十分重要的意义。
2 数值模拟
2.1 软件介绍
FDS (Fire Dynamics Simulator)由美国国家标准研究所(NIST: National Institute of Standards and Technology)建筑火灾实验室(Building and Fire Research Laboratory)开发的计算流体动力学软件,专门用于火灾动态模拟。FDS采用N-S方程,其主要内容为通过公式计算获得烟气、热传递的具体过程。FDS是火灾动态模拟的开源程序,其可靠性已得到大量实验验证。湍流数值模拟主要方法有直接数值模拟DNS (Direct Numerical Simulation)和大涡模拟LES (Large Eddy Simulation)。
1) 质量守恒方程
(1)
式中:
为泄漏气体密度,kg/m3;t为模拟时间,s;
为拉普拉斯算子;u为泄漏气体速度矢量,m/s。
2) 动量守恒方程
(2)
式中:P为压力,Pa;f为外力矢量,N;
为粘性矢量,
。
3) 能量守恒方程
(3)
式中:h为泄漏气体组分焓值,KJ/kg;q为气体单位体积释热速率,KW/m3;q为辐射热通量,KW/m2;
为耗散率,KW/m3。
4) 组分守恒方程
(4)
式中:Yi为第i种组分的质量分数;Di为第i种组分的扩散系数,m2/s;mi为第i种组分的单位体积生成率,kg/m3·s。
(5)状态方程
(5)
式中:R为气体常数,J/mol·K;T为温度,K;Wi为第i种组分的摩尔质量,kg/mol;W为混合气体摩尔质量,kg/mol。
2.2. 模型设置
数值模拟中,假设一直径500 mm的高压燃气管道发生破裂,产生一直径100 mm的喷射口,在喷射口前方垂直方向不同距离设置障碍物。在不同泄漏孔气体流速的条件下,分别在前方1 m、1.5 m、2 m、2.5 m、3 m处设置厚度为0.2 m的混凝土墙障碍物。在泄漏孔垂直方向分别设置温度切片,热辐射损失切片,甲烷浓度切片。由于泄漏孔前方设置有障碍物,当喷射火撞击到前方障碍物时会延墙面扩散,因此在墙面也分别设置温度切片,热辐射损失切片,甲烷浓度切片。为研究喷射火某一点的具体数据,在泄漏孔前方沿垂直方向布置一排热电偶和甲烷气体探测器,并且向上每隔0.3 m布置一排。详细工况见下表1。
3 结果与讨论
3.1 热辐射损失
喷射孔侧面垂直方向和前方障碍物墙面热辐射损失范围为−1900~0 kW/m3,如表2。喷射孔侧面垂直方向的热辐射损失最大值与孔–板间距和气体泄漏速率无明显关联。气体泄漏速率为30 m/s时,随着孔–板间距的增加,前方障碍物墙面热辐射损失值的范围逐渐变小,热辐射损失最大值变小,由于喷射火火焰未撞击到障碍物墙面,导致墙面有温度变化的范围较小,故热辐射损失变化范围较小。
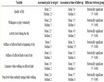
Table 2. Statistics of heat radiation loss for different hole-plate spacing (gas leakage rate of 30 m/s)
表2. 不同孔–板间距热辐射损失统计表(气体泄漏速率为30 m/s)
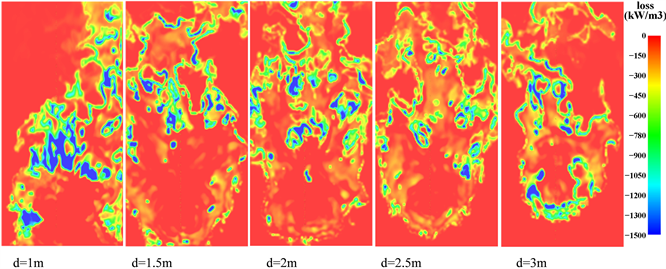
Figure 1. Distribution of heat radiation loss on the wall of obstacles with different hole-plate spacing (d) at a gas leakage rate of 60 m/s
图1. 气体泄漏速率为60 m/s时不同孔–板间距(d)障碍物墙面热辐射损失分布
当气体泄漏速率为60 m/s,不同孔–板间距障碍物墙面热辐射损失分布情况,如图1。随着孔板间距的增大,前方障碍物墙面热辐射损失变化范围分布逐渐变小,随着孔–板间距的增加,泄漏气体燃烧产生的喷射火撞击到障碍物墙面的范围逐渐减小,导致墙面的温度变化区域逐渐减小,因此热辐射损失变化范围分布逐渐变小。不同孔–板间距泄漏孔中轴线上泄漏孔与障碍物表面之间的热辐射损失分布情况,随着孔–板间距的增加,热辐射损失分布区域逐渐变大,且发生加大热辐射损失的区域逐渐变大。主要原因为甲烷气体由泄漏孔喷出后与空气发生卷吸,孔–板间距越大,卷吸的空气越多,产生的喷射火越大,发生温度变化的区域越大,因此发生热辐射损失的区域变大。图2为固定孔–板间距为2.5 m,不同泄漏速率障碍物墙面热辐射损失分布情况,随着气体泄漏速率的增加,障碍物墙面发生热辐射损失的范围逐渐变大,主要是因为随着气体泄漏速率的增加前方障碍物墙面的喷射火温度分布面积逐渐增大,墙面发生温度变化的区域逐渐变大,因此产生热辐射损失的范围逐渐变大。
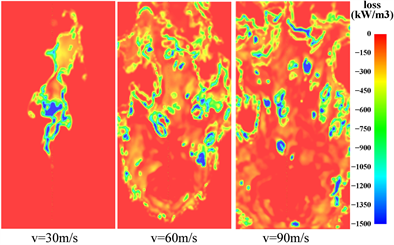
Figure 2. Distribution of heat radiation loss from obstacle walls for different gas leakage rates (v) at a front obstacle spacing of 2.5 m
图2. 前方障碍物间距为2.5 m时不同气体泄漏速率(v)障碍物墙面热辐射损失分布
3.2 甲烷浓度分布
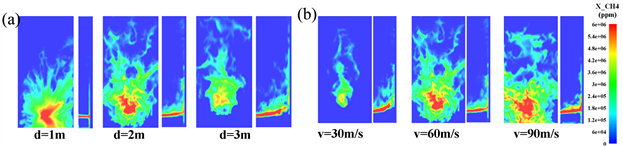
Figure 3. (a) Distribution of methane concentration at different hole-plate spacing (d) for a gas leakage rate of 60 m/s; (b) Distribution of methane concentration at different gas leakage rates (v) for a hole-plate spacing of 2 m.
图3. (a)气体泄漏速率为60 m/s时不同孔–板间距(d)下甲烷浓度分布;(b) 孔–板间距为2 m时不同气体泄漏速率(v)下甲烷浓度分布
前方障碍物墙面上,孔–板间距与甲烷浓度及分布范围成反比,如图3(a)。随着孔–板间距的增加,撞击到障碍物墙面上的甲烷气体逐渐减少,甲烷气体与空气发生的卷吸现象更加明显,甲烷气体与障碍物墙面发生的反射流现象减小。燃气喷射火焰厚度会随着孔–板间距的增大而增大。由于孔–板间距较小时大量甲烷气体从泄漏孔喷出后直接撞击到障碍物墙面,存在较多的未燃燃气,该部分未燃气体需撞击障碍物扩散后卷吸周围空气进行燃烧,温度会低于喷射火焰的最高温度,障碍物墙面的温度也会因此偏低。图3(b)为孔–板间距为2 m时不同气体泄漏速率下的甲烷浓度分布。前方障碍物墙面上甲烷浓度和分布范围与气体泄漏速率成正比。泄漏孔中轴线上泄漏孔与障碍物表面之间的甲烷浓度,随着气体泄漏速率的增加,撞击到障碍物墙面上的甲烷气体逐渐增加,甲烷气体与空气发生的卷吸现象减少,甲烷气体与障碍物墙面发生的反射流现象增加。因此,燃气喷射火焰厚度会随着气体泄漏速率的增大而减小。
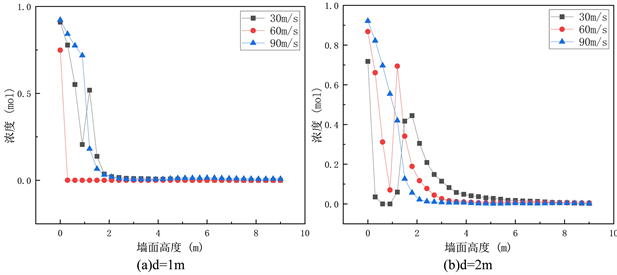
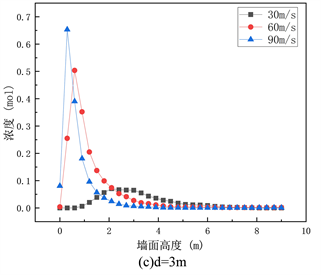
Figure 4. Variation of methane concentration on vertical wall for different hole-plate spacing (d)
图4. 不同孔–板间距(d)下竖直墙面甲烷浓度变化图
图4为前方障碍物间距分别为1 m、2 m、3 m时不同气体泄漏速率下的障碍物墙面甲烷浓度随墙面高度变化折线图。总体趋势为随着墙面高度增加,甲烷浓度减小,在1~2 m达到最大浓度,其原因为在甲烷到达更高的高度之前已经完全燃烧,可见喷射火的主要燃烧区域在墙面2 m的范围。图4(c)为孔–板间距为3 m时不同气体泄漏速率下的障碍物墙面甲烷浓度,泄漏速率为30 m/s时,甲烷浓度远低于最高值,证明在此情况下甲烷在到达墙面之前已完全燃烧,流速为60 m/s和90 m/s时剪完浓度呈现随着高度先上升后下降的趋势,说明前方障碍物过远,甲烷在撞击到墙面之前已发生向上抬升。
4. 结论
本文利用FDS火灾动力学模拟软件分析了不同气体泄漏速率和孔–板间距限制下射流火灾特性。以下是通过数值模拟得出的结论:
1) 随着孔板间距的增大,墙面热辐射损失变化范围分布逐渐变小;不同孔–板间距泄漏孔中轴线上泄漏孔与障碍物表面之间的热辐射损失分布情况,随着孔–板间距的增加,热辐射损失分布区域逐渐变大,且发生加大热辐射损失的区域逐渐变大。
2) 随着孔–板间距的增加,撞击到障碍物墙面上的甲烷气体逐渐减少,甲烷气体与障碍物墙面发生的反射流现象减小,孔–板间距与甲烷浓度及分布范围成反比;前方障碍物墙面上甲烷浓度和分布范围与气体泄漏速率成正比。泄漏速率为60 m/s和90 m/s时剪完浓度呈现随着高度先上升后下降的趋势,表明甲烷在撞击到墙面之前已发生向上抬升。
基金项目
重庆科技大学研究生创新计划项目(项目批准号:YKJCX2220702)、重庆科技大学研究生创新计划项目(项目批准号:YKJCX2220703)。