1. 引言
随着社会对环保要求的提高,以氟利昂为主的传统制冷技术面临着巨大的挑战,而室温附近的磁热效应因为其绿色环保、低噪声等在制冷技术上的潜力受到人们的广泛关注 [1] [2] [3] [4] [5]。在过去的研究中发现,含有重稀土元素的一级相变材料存在着较大的磁熵变,一直是人们研究的重点,但是这些材料同时存在着磁滞后大、可操作温区小的缺点;而二级相变材料的磁滞和热滞较小,这使其具有成为磁制冷材料的潜力 [6]。R-TM2(R = 稀土,TM = 过渡金属)型Laves相材料的磁热效应在理论及实验方面受到大家的广泛关注。在R-TM2型化合物中,RCo2型化合物又是其中研究最多的化合物 [7] - [16]。研究发现,ErCo2在42 K时表现出铁磁性到顺磁性转变特征,并显示出较大的磁热效应 [17]。由于磁热效应在居里温度附近才会出现最大值 [18],因此ErCo2极低的居里温度限制了其在室温制冷上的应用。本工作通过用Fe对Co进行替代使ErCo2合金的居里温度提升至室温附近,并且使其由一级相变转变为二级相变,提高了材料的实际应用潜力。
2. 实验方法
实验采用纯度为99.9%的稀土金属Er和纯度为99.99%的过渡金属Fe和Co。按Er(Co1-xFex)2 (x = 0,0.1,0.16,0.2)的化学配比将原料放入真空熔炼炉中,充入氩气后反复熔炼5次至样品均匀。为了补偿稀土金属在熔炼过程中的损失,配料时稀土金属Er质量过量5%。随后将熔炼均匀的铸锭放入高真空的石英管中并在退火炉中以973 K的温度退火7天并炉冷至室温。利用X射线衍射仪(XRD,型号为Panalytical Empyrean,CuKα)在室温下测量样品的晶体结构。用振动样品磁强计(VSM)测试样品的磁性,最大磁场为30 kOe。使用MATLAB编程软件处理数据,计算出Er(Co1-xFex)2 (x = 0.1,0.16,0.2)的熵变值。
3. 结果与讨论
我们在室温下对样品进行了X射线衍射分析,得到了样品的晶体结构。图1(a)为Er(Co1-xFex)2 (x = 0,0.1,0.16,0.2)系列粉末合金的XRD图谱,从图1(a)中可以看出,样品经过973 K的温度退火后呈现出单一的Laves相。图1(b)为Er(Co1-xFex)2 (x = 0,0.1,0.16,0.2)系列合金样品在440位置放大峰的XRD图谱,可以看出随着Fe含量的增加,衍射峰逐渐向较低的布拉格角移动,这是Fe的离子半径比Co的离子半径大所引起的 [19]。将样品衍射数据用Rietveld结构精修软件GSAS处理 [20],图2(a)~(b)分别为Er(Co0.84Fe0.16)2的XRD精修图谱、Laves相晶体结构示意图。图2(a)中“×”表示实验谱,红线表示拟合谱,蓝线表示强度差值,绿色竖条表示峰位。将样品的XRD数据进行精修后,得出样品的晶格常数为7.185 Å,材料的晶体结构为典型的立方MgCu2型晶体结构,空间群为Fd-3m。
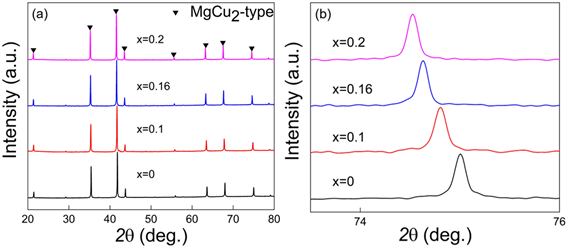
Figure 1. (a) XRD patterns and (b) the 440 peak of the alloys Er(Co1-xFex)2 (x = 0,0.1,0.16,0.2)
图1. Er(Co1-xFex)2 (x = 0,0.1,0.16,0.2)系列合金粉末样品的(a) XRD图谱和(b) 440峰图谱
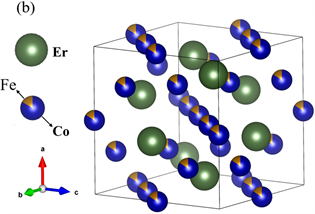
Figure 2. (a) Rietveld refined power XRD patterns and (b) the crystal structure of the Er(Co0.84Fe0.16)2
图2. Er(Co0.84Fe0.16)2的XRD精修图谱和Laves相晶体结构示意图
图3为200 Oe下Er(Co0.9Fe0.1)2合金的磁化强度随温度变化的曲线,内嵌图为材料的居里温度随成分变化的曲线。从图中可以看出,Er(Co0.9Fe0.1)2合金的居里温度大约在150 K左右。Laves相化合物的居里温度由3d-3d原子的交换能决定,受3d-4f原子杂化调节 [6]。随着Fe含量的增加,材料的居里温度由ErCo2的42 K增加到Er(Co0.8Fe0.2)2的338 K,并且在x = 0.16时,居里温度达到室温附近,如内嵌图所示。这说明Fe对Co的替换增强了3d-3d原子交换能。通过Fe的替换使Er(Co1-xFex)2合金的居里温度提高到室温附近,这让该合金在磁致冷领域有了实际应用的可能。
图4为Er(Co1-xFex)2 (x = 0.1,0.16,0.2)系列合金在居里温度附近的等温磁化曲线。可以看出,居里温度附近的曲线都很平滑,在最大磁场到达3 T时没有发生磁矩突变,即没有出现明显的巡游电子变磁转变 [21] [22]。
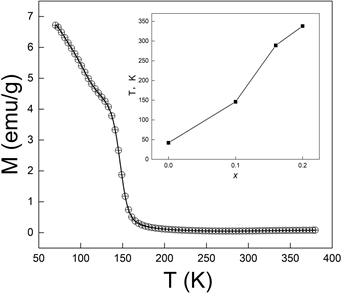
Figure 3. Temperature dependence of the magnetization for Er(Co0.9Fe0.1)2 at 200 Oe. The inset presents composition dependence of the Curie temperature for Er(Co1-xFex)2 (x = 0,0.1,0.16,0.2)
图3. 200 Oe下Er(Co0.9Fe0.1)2合金磁化强度随温度变化的曲线;内嵌图为材料的居里温度随成分变化的曲线
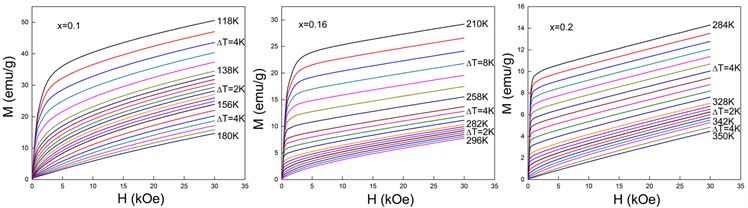
Figure 4. Magnetic field dependence of the magnetization for Er(Co1-xFex)2 (x = 0.1,0.16,0.2) near Curie temperature
图4. Er(Co1-xFex)2 (x = 0.1,0.16,0.2)系列合金在居里温度附近的等温磁化曲线
为了确定样品的相变类型,我们绘制了Er(Co0.9Fe0.1)2合金的Arrott曲线,其结果如图5(a)所示。根据A. Banerjee提出的判定标准,通过Arrott曲线的斜率可以来确定化合物的相变类型 [23] :若Arrott曲线表现有负的斜率或存在拐点,则该材料的相变类型为一级相变;若Arrott曲线斜率为正,则该材料的相变类型为二级相变。从Arrott曲线可以看出,Er(Co0.9Fe0.1)2合金的Arrott曲线斜率为正值,说明Er(Co0.9Fe0.1)2合金的相变类型为二级相变。通过过渡金属Fe对Co的替换,该系列化合物由一级相变转变为二级相变。
根据朗道理论,Gibbs自由能可表示为:
(1)
式中,T为时间;M为磁化强度,G0为无序相的自由能;H为磁场强度;A(T),B(T)和C(T)是与温度有关的常数。为了维持体系能量最小,
,状态方程可以表示为:
(2)
通过公式(2)可以得知,对Arrott曲线高场部分进行线性拟合后就可以得到系数A(T)、B(T)和C(T)的曲线,其中A(T)和B(T)的曲线已经画在图5(b)中。根据Inoue-Shimizu模型 [24] [25],系数A(T)代表材料的反转磁化率,并且A(T)在居里温度时取最小值;系数B(T)可以用来表征相变类型,在居里温度时如果B(T)为负值代表相变为一级相变,如果为非负值则代表材料的相变为二级相变。如图5(b)中所示,在居里温度附近A(T)出现极小值,并且对应的B(T)为正,这说明了Er(Co0.9Fe0.1)2合金的相变类型为二级相变。
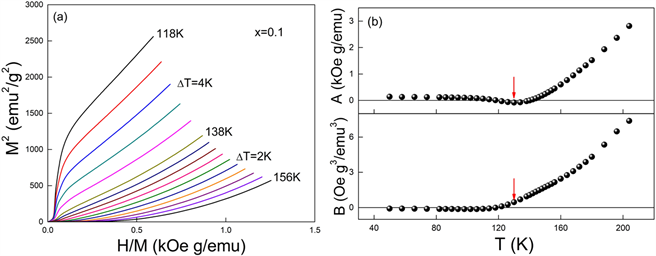
Figure 5. (a) Arrott plots of Er(Co0.9Fe0.1)2; (b) The fitted parameter A and parameter B as a function of temperature
图5. (a) Er(Co0.9Fe0.1)2合金的Arrott曲线;(b) 参数A和B随温度变化的曲线
我们计算了各个样品的绝热磁熵变。磁熵变跟磁场有以下的关系:
(3)
式中,ΔS是磁熵变,H是磁场,T是温度。根据Maxwell方程:
(4)
将(3)式换算成:
(5)
因此,对于在离散场和温度间隔测量的磁化强度,(3)式中的ΔS可以近似用下列等式表示 [26] :
(6)
其中Mi和Mi+1是在施加磁场为Hi的情况下,分别在温度Ti和Ti+1下测得的磁化强度。图6给出了利用公式(6)计算出的三个样品在不同磁场下的等温磁熵变。从图6可以看出,随着磁场的增加,三个样品的磁熵变逐渐增加,其中,Er(Co0.9Fe0.1)2的磁熵变值最高,在30 kOe大小的磁场下为2.834 J/kg·K,但是其峰值在120 K以下,不适合室温应用。Fe含量多时合金的熵变曲线与ErFe2类似,Fe少时合金的熵变曲线与ErCo2类似。通常情况下,可以在居里温度附近看到峰值,但是由于Er和Fe,Co的亚铁磁磁矩耦合,在Fe含量高时没有观察到峰值,其中具体的物理机制还有待解决。当x≥ 0.16时,材料的熵变值随着温度的降低而逐渐升高,并在一个较宽的温域保持了一个较大的熵变。因此该材料可以作为室温磁制冷材料的候选材料,这也提供了一种利用亚铁磁材料的相变获取宽温域磁制冷材料的新思路。
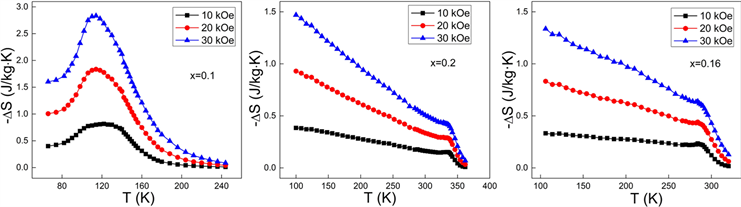
Figure 6. Magnetic entropy change vs temperature of the Er(Co1-xFex)2 (x = 0.1,0.16,0.2) compounds at different magnetic field
图6. Er(Co1-xFex)2 (x = 0.1,0.16,0.2)化合物在不同磁场下磁熵变随温度变化的曲线
4. 结论
1) 通过电弧熔炼和真空退火的方法成功制备了具有单一Laves相的Er(Co1-xFex)2(x = 0,0.1,0.16,2)系列合金。合金为ErCo2型立方晶体结构,空间群为Fd-3m。随着Fe含量的增加,晶格常数a逐渐增加。
2) Fe对Co的替代虽然降低了样品的磁熵变,但其相变类型由一级相变变为了二级相变,降低了磁滞和热滞,同时将居里温度提高至室温,扩展了合金的磁制冷温区,使样品具有了室温磁致冷的应用潜力。
3) 当x ≥ 0.16时,Er(Co1-xFex)2的熵变值随着温度的降低而逐渐升高,并在一个较宽的温域保持了一个较大的熵变。我们认为这一非常规的熵变曲线与Er(Co1-xFex)2中的稀土和过渡金属的亚铁磁耦合有关。这为今后的研究提供了一种利用亚铁磁材料的相变获取宽温域磁制冷材料的新思路。
基金项目
本研究受到了国家自然科学基金No.11475086项目的资助。